For those curious about some vocabulary and parameters used in our forecasts, below is a rudimentary introduction to meteorology and our forecasting criteria, as well as resources to better inform yourself of hazardous weather. If you find yourself more curious about specifics, feel free to email our staff!
Scales
We commonly refer to trends completely spanning the Earth as part of the Global scale, and trends occurring nationally as belonging to the Synoptic Scale. The Mesoscale defines smaller regions of activity generally occurring within a U.S. state, such as thunderstorms, and the Microscale pertains to short lived, very local phenomena occurring in distances less than a thousand feet.
Jet Streams and Pressure
The Jet Stream is a global scale phenomena, and it is a belt of high winds traveling overall from west to east that wraps around the globe. This is located in what is called the upper-level atmosphere, which means that it is located higher up in the atmosphere at altitudes where airplanes usually fly. We will sometimes talk about mid-levels and lower-levels, which as the name implies, pertains to conditions in the middle levels of the atmosphere, and lower levels of the atmosphere, within a mile or so of the surface. The Jet Stream serves to steer most of the weather we experience in the United States. The Jet Stream will follow the boundaries of warm and cool air masses, so it is often not a straight west-to-east path, instead, dipping up and down across lines of latitude. These fluctuations in the jet stream can be labeled as troughs and ridges. In the U.S., troughs are associated with colder air further north moving south, and ridges are associated with warmer air further south moving north. Terms you will sometimes hear in our technical discussions are shortwaves and longwaves. These are used to describe the size of troughs. Jet streaks are small, intense bands of winds located within Jet Streams, which we will sometimes mention when covering a forecast for severe weather, as specific regions within jet streaks can promote strong storm development.
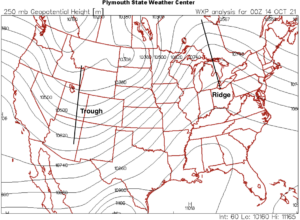
An example of an upper-level map with varied flow. A trough and a ridge are labeled. Credit to the Plymouth State University.
Pressure systems are likely one of the most common terms used within our forecasts, and are usually synoptic-scale phenomena. High pressures are regions of sinking air, which are usually associated with calm, clear weather (hence, ‘High and Dry’). Low pressures are regions of rising air, and are typically associated with precipitation and inclement weather. The mid-latitude cyclone is a common form of low pressure systems that affects us nationally. In typical mature mid-latitude cyclones, leading ahead of the system will be a warm front, which serves as the boundary between a cool, but often humid airmass, and moist, warm airmass. When a warm front passes, colder air will be pushed out, replaced by warmer and more humid air. Trailing below the mid-latitude cyclone is also a cold front, which is another boundary between the warm, moist air, and colder (but often drier) air. When a cold front passes, the warmer air is pushed out by colder air, and typically, thunderstorms will follow this line.
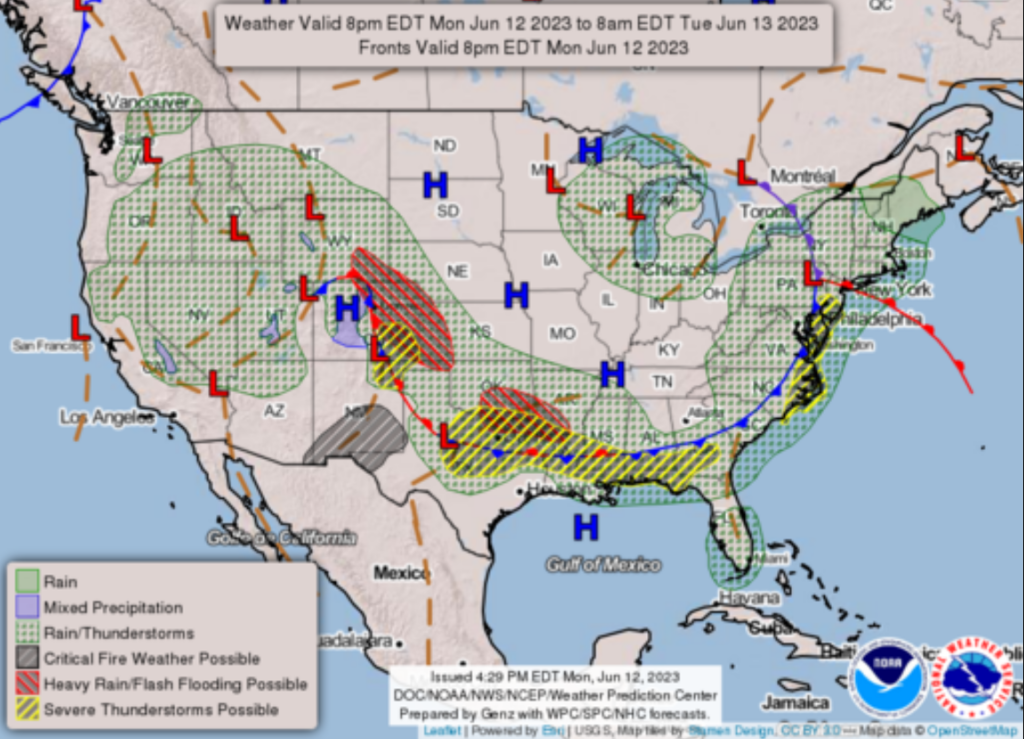
An example of a mature mid-latitude cyclone on the Eastern Seaboard. Credit to the Weather Prediction Center.
Thunderstorms
Thunderstorms are a mesoscale phenomena on their own, but synoptic scale conditions can directly lead to their development. When we forecast a thunderstorm, a few parameters must be kept in mind. To form clouds, air must rise. To make air rise, some level of forcing must be enacted, and fronts can serve as this forcing, as pushing two different masses of air together can force air to move upwards. Warmer air can hold far more moisture (in the form of invisible water vapor) than colder air, so when warm air is forced to rise, it moves into regions of lower pressure aloft, and expands and cools. The now colder air cannot hold as much moisture as it previously could. Therefore, this water vapor in this air is often forced to condense, which is why we have clouds. When the cloud liquid (or ice crystals) grow large enough, they can be pulled downward toward the Earth as precipitation.
The key ingredient that will make a thunderstorm instead of a rain shower is a parameter called instability. Instability is the tendency for air to continue rising after it has been forced upwards. Strong, unstable environments can be found within high temperature and high moisture environments at the surface with much cooler air aloft. When the forcing mechanism reaches this area of instability, towering cumulonimbus thunderstorm clouds can form.
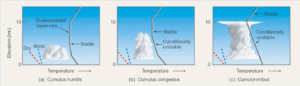
More unstable environments lead to stronger storms. Credit to Colorado University.
Severe Thunderstorms
From the National Weather Service, a severe thunderstorm is classified by having hail 1-inch or greater in diameter, wind gusts greater than 57.5 mph, or a tornado. When we forecast for a severe thunderstorm, we will look to see how unstable the air within the region is, as well as how the winds are behaving at different levels of the atmosphere. When winds either travel at different speeds or different directions at different heights, this is called wind shear. Among many things, wind shear can shape thunderstorms into different “modes”, such as QLCS (Quasi-linear-convective-systems) that form in a line, typically along a cold front, or supercells, classified by their rotating updraft, which can produce tornadoes. Our severe forecasts will follow a similar color system as the Storm Prediction Center, with yellow being a slight risk of severe thunderstorms, orange as enhanced, red as moderate, and pink as high. Pay attention to when even a slight risk of storms is issued, as the chance for damaging wind, hail, or even a tornado is possible.
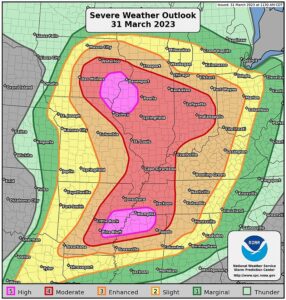
An example of the SPC’s categorical severe weather outlook. Credit to the Storm Prediction Center
Winter Weather
What is Winter Weather?
Winter weather can follow along mid-latitude cyclone systems, but the conditions are vastly different. Winter weather can encompass multiple types of liquid and frozen precipitation, namely snow, sleet, freezing rain, and rain. For it to snow, the lower levels of the atmosphere must have a wet bulb (NOT air temperature) below 32 degrees Fahrenheit for the snowflakes to remain as hexagonal ice crystals. Sleet occurs when an area of warmer air melts the snowflake into a raindrop, but the raindrop refreezes completely within sub-freezing air above the surface. These appear as ice pellets when they hit the ground. Freezing rain occurs when the wet bulb temperature above the surface is warmer than 32 degrees Fahrenheit, but a vertically shallow layer of air near the surface is below freezing. This precipitation will fall as rain, but then freeze on or soon after contact with the ground. Should the wet bulb temperature profile remain above freezing consistently, what originates as snow will melt and stay as rain to the surface.
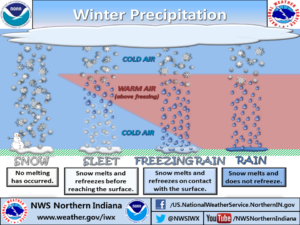
A cross-sectional example of how warm air can transform snow into different precipitation types. Credit to NWS Indiana
Forecasting Winter Weather
Winter weather remains one of the most challenging aspects of forecasting, largely due to the narrow temperature thresholds that determine the four main types of precipitation. Understanding where winter weather is likely to occur begins with recognizing its association with common weather features. In the Midwest, the midlatitude cyclone is often the primary driver of winter weather. Its interactions with high-pressure systems play a critical role in determining the geographical extent of winter conditions. These interactions are shaped by the balance of warm and cold air advection, as well as the availability of atmospheric moisture. Refer to the left figure for an illustration of typical weather patterns that contribute to winter weather.
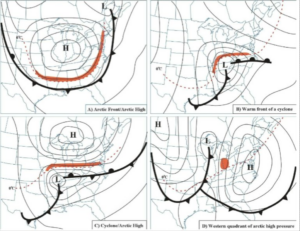
A four-panel figure of different pressure systems and their fronts (black and bold), and the relative location of where winter weather may occur (orange).
When using forecasting models, distinguishing between types of winter precipitation often begins with analyzing the 5400-gpm line (commonly referred to as “540”) on a 1000-500 mb thickness map. Geopotential thickness is directly proportional to the average virtual temperature between two atmospheric levels: warmer air results in a thicker column, while colder air produces a shallower column. Consequently, regions with heights greater than 5400 gpm typically experience rain, whereas heights below 5400 gpm are more likely to produce snow. Identifying this boundary on a map helps forecasters determine where precipitation is likely to transition from rain to snow within a region.
In addition, analyzing temperature advection at the 800 mb and 925 mb levels is critical for differentiating precipitation types. For example, warm air advection ahead of a warm front can lead to freezing rain, as rising warm air overlays a surface layer of subfreezing temperatures. Conversely, cold air advection can result in rain transitioning to snow, sleet, or freezing rain, depending on the depth and intensity of the cold air. Therefore, one of the most valuable tools for identifying differential advection across atmospheric levels is the Skew-T log-P diagram, which provides a detailed vertical profile of the atmosphere.
Forecasting snowfall amounts often utilizes snowfall ratios. Traditionally, the “10:1 ratio” (10 inches of snow equals 1 inch of liquid water) has been a standard reference. However, this ratio can vary significantly depending on atmospheric conditions. For example, when the temperature throughout the layer from the surface to the cloud hovers near freezing, snowfall ratios tend to decrease, whereas a deep, cold atmospheric layer can lead to much higher snowfall ratios
Winter Weather Safety
Understanding the National Weather Service’s criteria for winter weather alerts is essential for assessing the potential threat posed by a winter weather event. For the Charleston, WV Forecasting Office, Watches are issued when forecaster confidence in meeting criteria reaches 50%, typically 12 to 72 hours before the onset of wintry conditions. Warnings and Advisories are issued when forecaster confidence reaches 80%, usually within 24 hours of the event’s onset. These criteria may be adjusted based on the anticipated impacts of the event. Below are some of the alerts that may be issued, along with their specific criteria.
Blizzard Warning
- Sustained wind or frequent gusts of 35 mph or higher, AND visibility less than 1/4 mile in snow and/or blowing snow for 3 hours or longer.
Ice Storm Warning
- Damaging ice accumulations of 1/4″ or more.
Winter Storm Watch/Warning
- Snowfall of 4-6″ or more, event-driven up to a 48-hour period, OR sleet accumulation of 1/2″ or more in 24 hours.
Winter Weather Advisory
- Snow, blowing snow, ice, sleet, or a combination of these wintry elements is expected to cause winter driving conditions and difficult travel.
Cold Weather Advisory
- Temperatures and/or wind chills at -5 degrees or lower.
Extreme Cold Watch/Warning
- Temperatures and/or wind chills at -15 degrees or lower
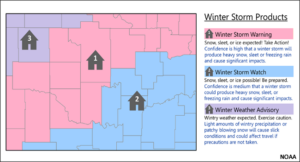
Infographic by the National Weather Service defining the hazards contributing to the issuance of different winter weather alerts.
In addition, local Emergency Management agencies may issue Snow Emergencies to warn the public about the hazards of driving under current conditions. These emergencies are categorized into the following levels:
Level 1: Roadways are hazardous with blowing and drifting snow. Roads may also be icy. Motorists are urged to drive very cautiously.
Level 2: Roadways are hazardous with blowing and drifting snow. Roads may also be very icy. Only those who feel it is necessary to drive should be out on the road.
Level 3: All roadways are closed to non-emergency personnel. No one should drive unless absolutely necessary.
Before a winter storm arrives, ensure you are stocked with essential survival items, including a first aid kit, water, non-perishable food, heavy clothing, and blankets for insulation. Additionally, have batteries, chargers, and flashlights readily available. For your vehicle, keep a full tank of gas and equip it with jumper cables, shovels, brushes, a spare tire, and an emergency kit. If you have pets or livestock, move them to sheltered areas or indoors, and ensure they have sufficient water and fresh food.
If you are caught outside during a winter storm, seek the nearest shelter and cover any exposed skin to avoid frostbite. If driving, reduce your speed significantly and ensure you have charged devices and an emergency kit. Avoid travel unless absolutely necessary. If you are indoors, remain there. If the heat goes out, close off unnecessary rooms to conserve warmth and insulate your living area as much as possible. After a winter storm, continue to stay off the roads until conditions improve, as hazards such as flooding from snowmelt or downed power lines may persist.
Below are several helpful resources to deepen your understanding of winter weather preparedness and safety strategies.
https://www.weather.gov/safety/winter
https://www.weather.gov/rlx/criteria
https://www.weather.gov/arx/wintersafety
https://weathersafety.ohio.gov/weather-safety/winter-weather
https://ready.ohio.gov/are-you-ready/winter-storms
How Scalia Lab Forecasts
Our forecasts listed on our icon cards span from 0-48 hours. Typically, this information will be more specific and more accurate than our long range forecasts, which encompass 48-108 hours. Our forecast discussions will be geared for the general public, though those wanting a more in-depth and lengthy forecast can read our technical discussions. Our color cards will often convey levels of cloud cover, as well as severe risks. The icons are a snapshot of the weather to come, much like you would see on your weather app. We aim to publish forecasts at approximately 8 AM and 8 PM, as well as provide live-updates to severe or winter weather through our social media outlets. Our area of coverage extends from Ohio University to our neighbors around Southeastern Ohio.